Today is a great day for particle physics, as a discovery has been announced by the LHCb collaboration. A new particle has indeed been observed in LHC data.
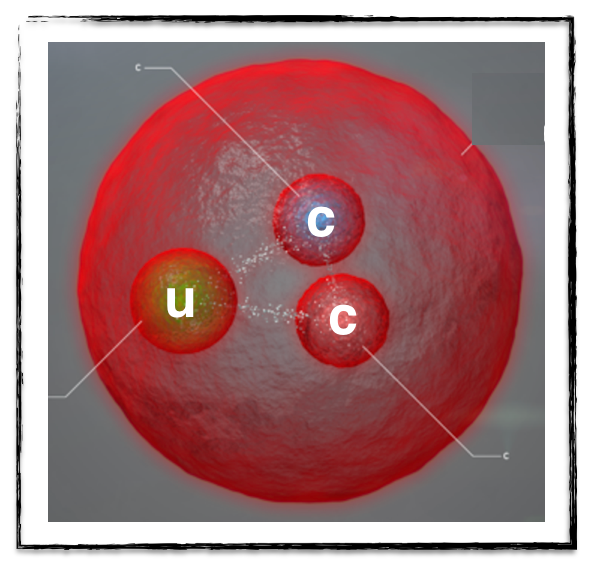
[image credits: CERN]
In a couple of previous posts, I already mentioned how well the LHCb experiment of the LHC is doing. And today, once again, LHCb surpassed all expectations: it observed a new particle made of two charm quarks (c on the picture on the left) and one up quark (u on the picture on the left).
Such a particle is named a Ξcc++, where there is in principle no spacing between the ++ and the Ξ symbol, but markdown is what it is…
It is also more or less convenient to pronounce: ‘ksi - see see - plus plus’.
The double ‘c’ stands for the fact that there are two charm quarks involved, and the double ‘+’ indicates that the electric charge is of 2 units.
Who told anyone that scientists were good in finding names? ;)
THE LHCb EXPERIMENT IN A FEW WORDS
It is good to mention, once again, some words about the apparatus that has made the discovery I want to talk about possible. The image below shows the LHCb detector being assembled in its cavern around the collision point of the two LHC beams.
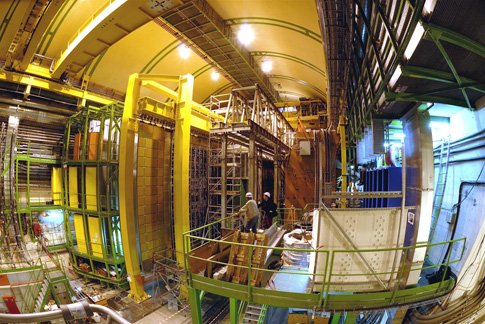
[image credits: LHCb @ CERN]
The LHCb detector has been built to study a very specific type of particles, that have the property that they are generally produced in a configuration where they stay close to the LHC beams.
As a result, the LHCb detector has been adapted and is very good in investigating what is going on along the LHC beam line.
It can be seen as a huge apparatus made of smaller subdetectors, that are aligned along the beam line starting from the collision point. This is very similar as when one aligns books in a bookshelf.
Each book (or subdetector) is dedicated to explain one feature of one or more particle species, and by putting all the information together, physicists are capable to reconstruct what happened in the collisions.
COMPOSITE STATES MADE OF QUARKS
At the beginning of this post, I mentioned that the new particle that has been discovered is made of three quarks: two charm quarks and one op quark.
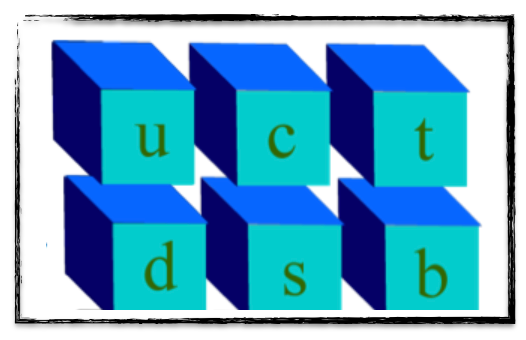
[image credits: homemade (from well spread stuff)]
The Standard Model of particle physics contains six species of quarks, that are known as the up (u), down (d), strange (s), charm (c), bottom (b) and top (t) quarks.
In addition, one can add to that list the corresponding antiquarks: each quark is always mapped with an antiparticle of the same nature.
Let us forget about the top quark. It is special and so heavy that it actually decays too fast for being able to form any composite state. We concentrate instead on the five other guys.
As I detailed it in this older post, quarks and antiquarks can form a composite particles by virtue of the strong interaction.
Such a composite state is the particle that has been discovered.
BARYONS AND THE NEW Ξcc++ PARTICLE
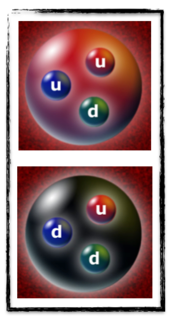
[image credits: everywhere on the web]
Quarks can in particular form systems called baryons.
A baryon is made of three quarks, like for instance two up quarks and one down quarks (uud) or two down quarks and on up quark (ddu). Those are in fact the well known proton and neutrons, as shown on the picture on the right.
Whilst any combination of quarks is in principle possible, as predicted by the theory, many possibilities, in particular those including two c quarks, have never been observed.
The Ξcc++ is one of those missing links, made of two charm quarks and one up quark.
HOW TO OBSERVE THIS NEW Ξcc++ PARTICLE
In order to conclude to the existence of this state, LHCb experimentalists were tracking the signature it would have left once produced. The Ξcc++ is indeed unstable and therefore leaves tracks in the detector when it decays.
We can predict the number of boring collisions yielding to the same signature but when ignoring any possible effect induced by the Ξcc particle, and we can also predict what is going on if the Ξcc particle exists.
The rest is a matter of comparing data to theory predictions and to tell which hypothesis accommodates data best, and at which confidence level,
[image credits: the LHCb publication on the discovery]
The signature that has been probed is illustrated on the above picture.
One of the charm quarks (on the bottom of the figure) of the Ξcc is converted into a strange quark (s) and a up-antidown (u dbar) pair.
Moreover, two quark-antiquark pairs (u ubar and d dbar) are created from the vacuum.
The nine produced quarks are then reorganized into one baryon (the Λc+ guy) and three mesons (the two π+ pions and the K+ kaon).
The quark content of this various particles can be guessed from the picture. The key point is that the four particles on the right can be precisely detected and identified.
THE OBSERVATION
From the four particles making the searched signature, one can reconstruct the initial state and study its properties, in order to be sure that we are in the presence of a new particle.
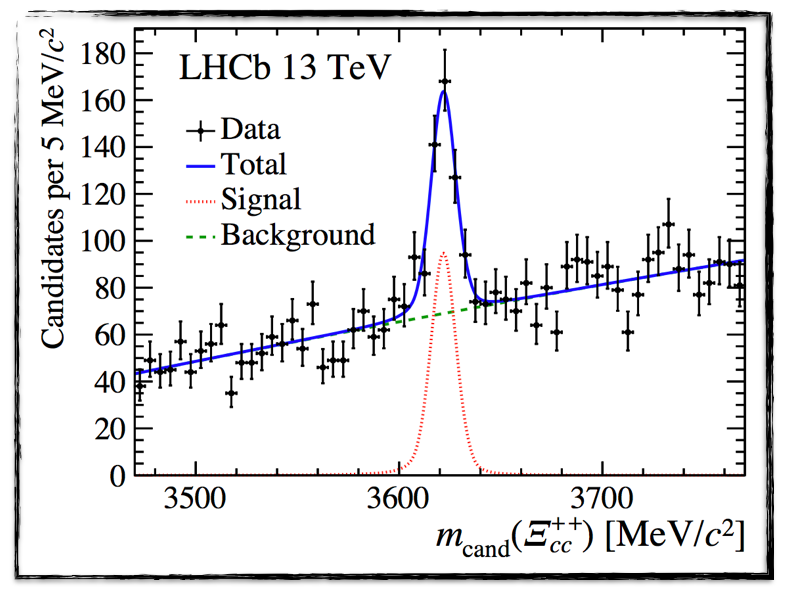
[image credits: the LHCb publication on the discovery]
This is illustrated on the picture shown on the right, where one can see what is expected without the presence of the Ξcc (green dashed line) and with the presence of the Ξcc (solid blue line). The black crosses are the data point.
We have thus a new particle here, and there is no way that the data is compatible with the background-only hypothesis.
In other words, the green line cannot agree with the black crosses and there is in fact less than one chance out of thousands of billions that nature cheats on us.
In addition, this discovery is compatible with signs arising from previous experiments (SELEX and the run 1 of the LHC) but where the confidence level was not high enough to claim anything.
SUMMARY AND REFERENCES
In this post, I summarized one of the announcements made at the EPS-HEP conference earlier this week. The LHCb collaboration of the LHC has announced the discovery of a new particles made of two charm quarks and one up quark, the so-called Ξcc++ particle.
This is very exciting as this consists of the experimental confirmation of a prediction that has finally been observed, and that will allows physicists to further study the theory of the strong interactions.
More information can be found: