In terms of the discovery of new phenomena, one expects the most from the ATLAS and CMS experiments at the LHC, the two general purpose experiments that discovered the Higgs boson in 2012. The detectors are indeed designed in such a way that they are perfect hunters for new phenomena.
However, not a single sign of anything non standard have been seen by ATLAS and CMS so far. There are however other experiments around the LHC accelerator, that are as interesting in terms of discovery potential.
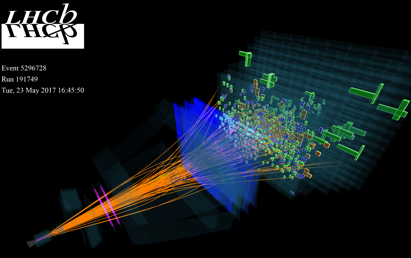
[image credits: LHCb @ CERN]
One of them is named LHCb, and LHCb data actually contains a bunch of puzzling anomalies that leave the physics community without any answer so far. Those belongs to the domain of flavor physics, that I will discuss today, together with the LHCb experimental setup.
For the anomalies themselves (that are potential signs of new particle physics phenomena), I will write a dedicated post later on. This article is indeed already long enough.
One typical event recorded by the LHCb detector (and that could have yielded the observed anomalies) is shown on the right. This is a real event following a collision that happened about a month ago (on May 23rd) at the LHC, when the 2017 data-taking period has restarted.
FLAVOR PHYSICS
Flavor physics has nothing to do with cooking or taste, but has actually a well-defined meaning in particle physics. As I depicted it in one of my very first post on Steemit (here), the Standard Model of particle physics contains 6 species of quarks named the down, up, strange, charm, bottom and top quarks.
In our jargon, we are actually also speaking of six flavors of quarks. And now one can easily guess what flavor physics is about.
More precisely, this consists of the study of the quarks, and how they actually mix. Standard Model processes indeed allow quarks to change nature, starting from one flavor and ending into another one. This results from the weak interactions that they are sensitive to.
Such flavor-changing processes can be described by a total of four parameters. Those parameters are probed via many measurements investigating the properties of various composite states made of quarks.
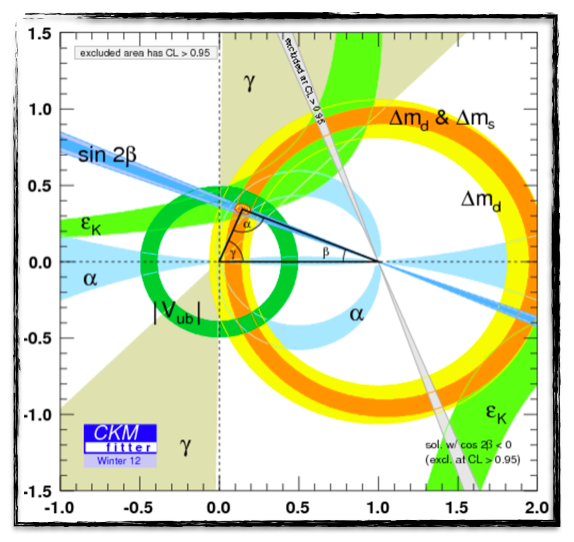
[image credits: CKMfitter]
In the figure shown on the left, we observe a triangle that summarizes an important result: all the flavor measurements heavily agree with the Standard Model.
The upper apex of the triangle is indeed well determined, at the intersection of all the colored regions stemming each from a particular measurement.
The fact that this works so well is another of the great successes of the Standard Model.
However, there are anomalies, in particular when heavy flavor quarks like b-quarks (another word for bottom quarks that are also known as beauty by the way) are involved…. But this is for later this week.
THE TOP QUARK
The heaviest quarks, the bottom and the top quark, have received a lot of interest by energy physicists.
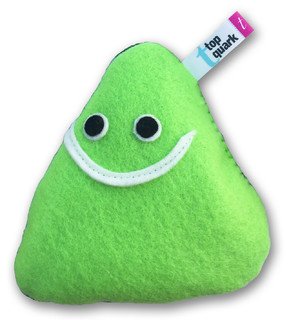
[image credits: the particle zoo]
The top quark, whose representative plush is shown on the right, is so heavy that it will decay into a bottom quark and a weak boson before being able to do anything else.
It is actually the heaviest of all particles of the Standard Model of for this reason, it is believed as a perfect laboratory for probing for new phenomena.
In terms of energy, the mass of the top quark is very close to the energy scale of electroweak symmetry breaking. In other words and more crudely, we carefully study the possible connections between the top quark and the Higgs boson to which it couples very strongly.
While the top quark could be the topic of a post on its own, I will stop here and instead focus on b-quarks whose properties exhibit non-understood anomalies that are cool to chat about.
THE BEAUTY AND THE MATTER-ANTIMATTER ASYMMETRY
The bottom quark (its symbol b also stands for beauty, as I said abpve) is not as heavy as the top quark, its mass being actually much smaller (1/45 times the top quark mass), but large enough so that it has an interesting phenomenology different from the one of the other lighter quarks.
Bottom quarks do not exist freely in nature. They rapidly form composite states, like mesons, and the latter can be detected. Those states are unstable and short-lived, and we can hence observe them flying a little bit before decaying.
At the Large Hadron Colliders, gazillions of b-quarks are produced. They then hadronize, i.e., form composite states by virtue of the strong interactions. The decay length of these composite states is large, so that we can follow their decay in the detector and measure their properties.
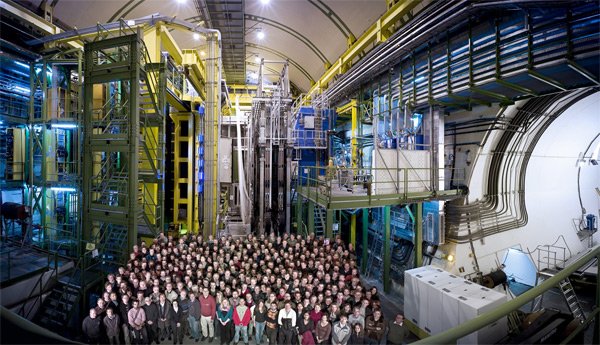
[image credits: LHCb @ CERN]
One of the key idea pursued, in particular at the LHCb experiment, is to study both the b-quark and b-antiquark. A careful comparison of the decay properties of the corresponding composite states may yield hints about why nature has preferred matter over antimatter.
The LHCb experiment has been designed to collect the largest amount of relevant data ever recorded. A picture of the LHCb detector (and of its the entire collaboration) can be found on the left.
Just to write down some numbers, LHCb involve about 700 scientists from 52 institutions from all around the world.
THE LHCb EXPERIMENT
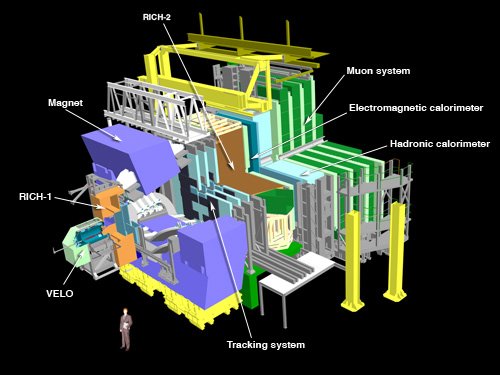
[image credits: LHCb @ CERN]
A representation of the LHCb detector is shown on the right. While the ATLAS and CMS detectors are onion-like detectors, LHCb is different.
To recap a little bit, an onion-like detector is exactly like an onion. It is made of several layers organized on top of each other, and each layer has a specific role (measuring tracks, energy, etc.).
LHCb is not like that for a very good reason: it has been built to study B mesons, that are composite states made of bottom quarks (see above). Those B mesons have the property that they stay close to the beam line of the LHC.
The detector is thus adapted to this feature, and aims to study what is going on along the beanline. It is 20 meters long and 4500 tons heavy, and the LHCb subdetectors are aligned one after the other, along the beamline. As sometimes referred to, as books on a shelf.
This configuration is very appropriate for B mesons study. Each of the LHCb subdetectors then focuses on a given type of properties of the particles produced in LHC collisions, so that tLHCb is very good in getting the particle identities, trajectories, energies, etc.
SUMMARY AND REFERENCES
In this article, I have reviewed what we meant, in particle physics, by flavor physics. I briefly discussed top and bottom quarks, and why an entire LHC collaboration, LHCb, is dedicated to the study of particles made of b-quarks (generically known as beauty).
The only signs we have today about new phenomena at the LHC are actually connected to b-quarks. I will dedicated an entire post to this in the next few days.
More information is available here: