Although I am a particle physicist, which is also known as a high-energy physicist, a large fraction of my research work focuses on the problematics of dark matter.
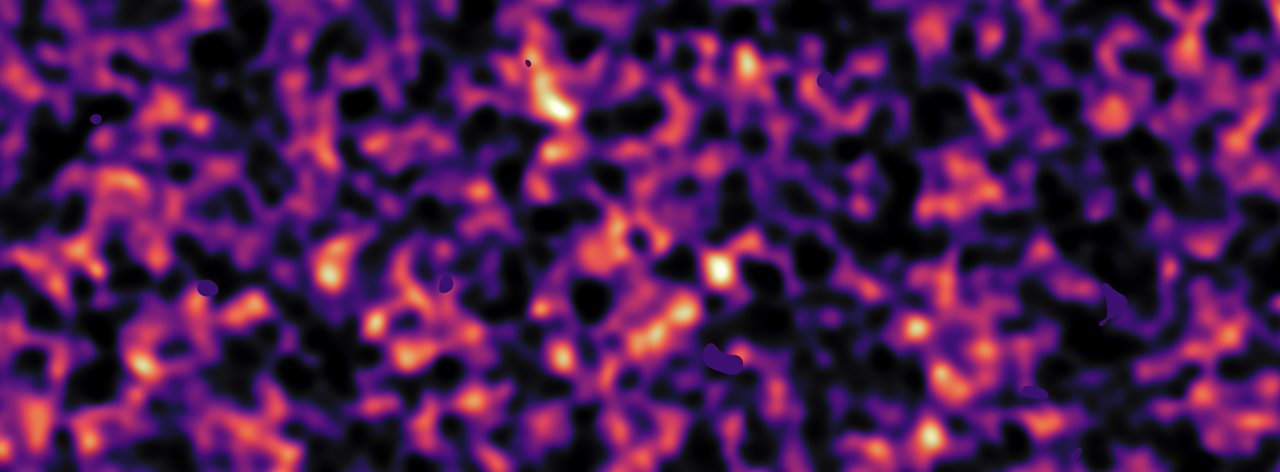
[image credits: Wikipedia]
A good question that may be asked for, is why the hell would someone interested by the smallest bits of matter may learn something from what is going on at the scale of the universe.
The answer is actually very simple and is based on only two assumptions.
With this article, I will try to explain why dark matter is important for particle physics, and I will focus, as an appetizer before the grande finale, on giving details on these two assumptions.
THE ORIGINS OF THE IDEA OF DARK MATTER
The first of these assumptions that I have mentioned above requires relies on the correctness of the dark matter paradigm. This is a very strong assumption, which I have already discussed in the past and that I will further discuss in this section.
As a good starting point, it is interesting to come back to where the dark matter idea is originally coming from. It is old, very old: it is already 85 years old.
The origins of the story comes from observations made in 1933 by Fritz Zwicky, who observed what was going on in a cluster of galaxies named the Coma Cluster.
Zwicky was actually not the first one to suggest the idea of dark matter, but he is the first one who brought on the table an indirect evidence of its existence.
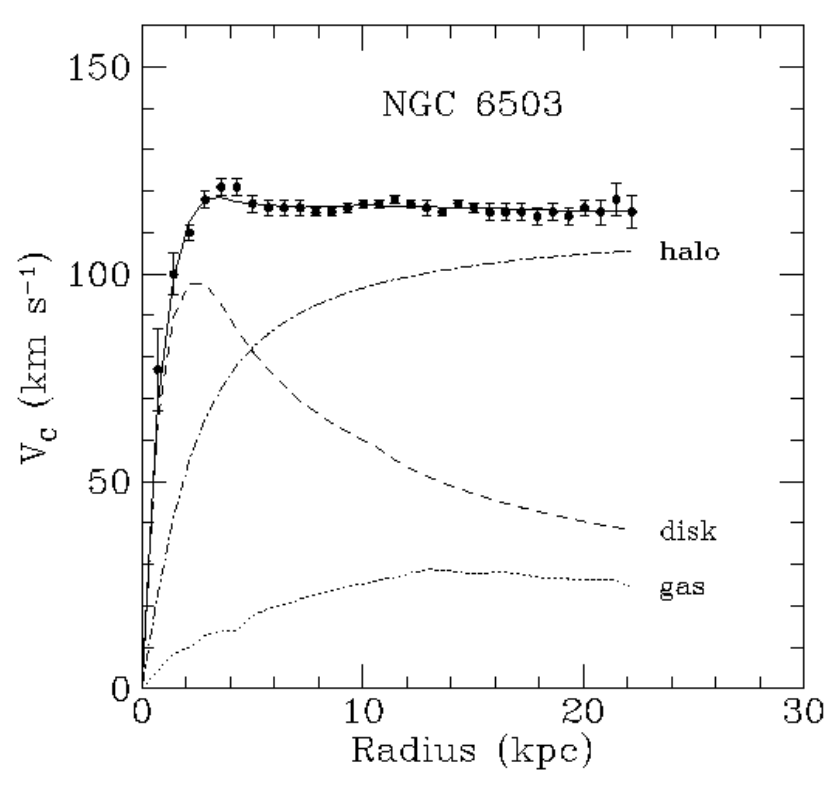
[image credits: arXiv]
In a few words, Zwicky observed the circular motion of stars and gas in a distant galaxy, as a function of their distance from the galactic center.
Moreover, on the basis of the mass of these visible stars and gas and with the help of Newtonian dynamics, predictions can be made for the quantities being observed.
An illustration of the results is given in the picture on the left. The predictions consist of the ‘gas’ line, whilst the data are the squares.
A strong disagreement can be observed, which has been in the meantime confirmed by many independent measurements.
There are two generic solutions in order to restore agreement between theory and data.
Either we change how gravity works at large distances, or we add matter that interacts gravitationally but that is invisible (or dark).
Both options are still viable today and researchers are working on both. I will focus on the dark matter option. which is the one I prefer.
OTHER INDIRECT EVIDENCE FOR DARK MATTER
But that is not all the story concerning the current evidences for dark matter. More data is available, and dark matter is capable to accommodate a lot of data.
This is why dark matter is still very much studied today as a very serious hypothesis to explain what is going on in the universe.
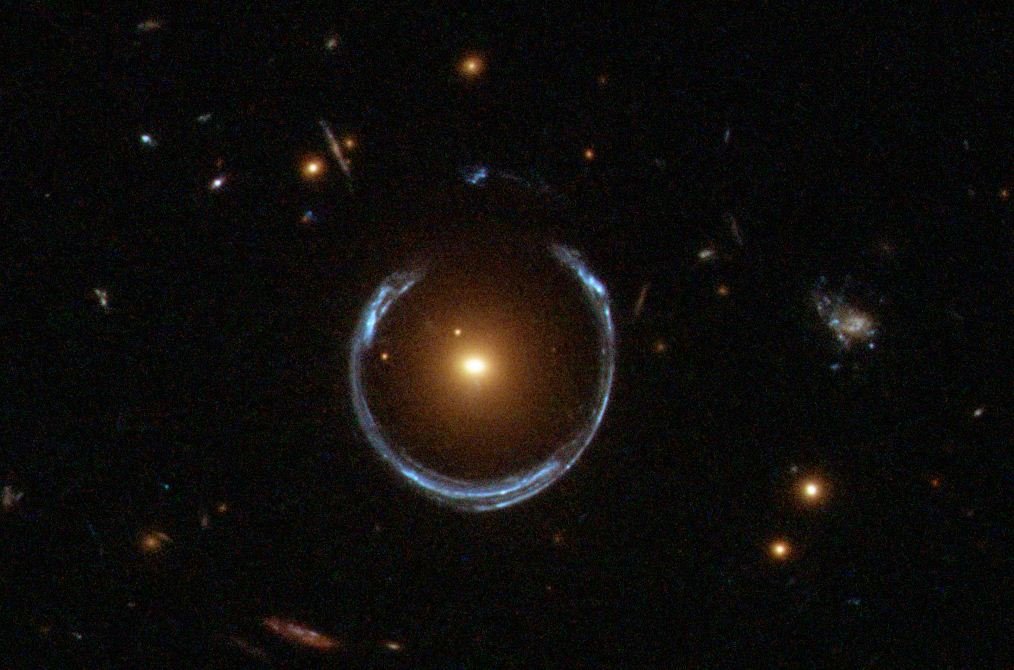
[image credits: Wikipedia]
Let us list a few of these evidences.
On galactic scale, one can mention gravitational lensing, one of the predictions of general relativity (illustrated by the picture on the right).
Matter bends the trajectory of light between an emitter source and the observer (i.e., us). Studying how light is bended allows us to go back to the amount of matter that bended the trajectory, which consists another evidence for a missing mass.
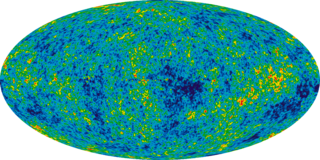
[image credits: Wikipedia]
Another indication of dark matter may come from the cosmic microwave background. The latter is super well measured by in particular the Planck experiment (as shown in the picture on the left).
The anisotropies that can be observed on the picture can be traced back to test cosmological models, and thus indirectly to dark matter which is an important component of many cosmological models.
BEYOND THE STANDARD MODEL
Now it is time to move back to particle physics.
The second of the above-mentioned assumptions concerns the fact that the Standard Model of particle physics, which is one of the most tested theories of all time and which survives all tests so far, is only the visible part of the ultimate theory describing the world of the elementary particles.
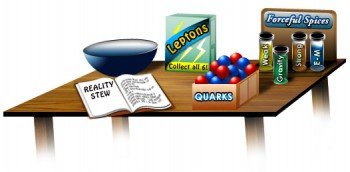
[image credits: The Particle Adventure]
There are indeed strong hints yielding physicists to think that the Standard Model is not this ultimate theory of nature.
Just to quote a few of them, one can mention the unification of the fundamental interactions, gravity, the large hierarchy between the strength of gravity and of the other interactions, etc.
I have written an entire post on this topic in the past, which can be found here. So for more information, I am lazily referring to this post :)
There is actually a huge field of research focusing on the possible theories describing the microscopic world, but beyond the Standard Model.
In a few words, researchers investigate what are the possible signatures of any theory that can be seen as a more fundamental extension of the Standard Model, how such a theory could be seen in past, present and future experiments, and what novel phenomena should be expected or could be used for unraveling the true nature of the entire particle physics iceberg..
MERGING THE INFINITELY BIG AND SMALL
Of course, constructing a theory describing how the elementary particle speak with each other is not too complicated, at least for someone who studied that area of physics.
The basic ingredients are the same as those necessary for building the Standard Model: quantum field theory, special relativity, gauge symmetries, etc… Those are a lot of big words, but they are not very important for the discussion.
What is important is that nothing really fancy is necessary. Of course, once in a while a new ideas appear, but such ideas can always be linked to the same basic principles underlying the Standard Model.
What is complicated is that one must construct viable theories with respect to data. Let us focus on an example, the theory ‘A’.
Let us assume that I am a big fan of a specific theory named ‘A’. If ‘A’ predicts a spectacular signature that should have already been observed in experimental data from the last ten years, ‘A’ must go into the bin.
No discussion. Any realistic theory must agree with data. And this is where dark matter comes into the game.
Many theories extending the Standard Model contains a particle that can play the role of dark matter. As we have a dark matter candidate, this dark matter candidate must not contradict astrophysical and cosmological data..
This is why astrophysics and cosmology are important for the construction of theories potentially extending the Standard Model!
SUMMARY AND REFERENCES
With this article, I have tried top summarize why astrophysics and cosmology matters for particle physics. In short, I have tried to motivate why many researchers in particle physics think the universe is dark, and why it matters for particle physicists tracking new phenomena.
(I must admit I am proud of this last sentence :p )
To go one step further: I have tried to motivate why dark matter is now one of the central stone of the current high-energy physics research program.
From more information, I suggest the following readings:
- The article where Zwicky studied the Coma cluster is available here.
- The wikipedia page is pretty accurate and contains many many references. I am not a big fan of referring to wikipedia, but this time, an exception is in order. See here and references there in.
- For the reasons why one should go beyond the Standard Model of particle physics, I am self-citing myself with my old steemit article.