Ten days left, and 20 steemSTEM members will meet at CERN, the largest high-energy physics facility in the world. Through this meeting, we will bring STEEM down inside the Large Hadron Collider and demonstrate that steemSTEM can push the frontiers further.
For more information about our meetup, please have a look here.
This also means that I have only 10 days left to finalize my introduction to particle physics…
Challenge accepted ;)
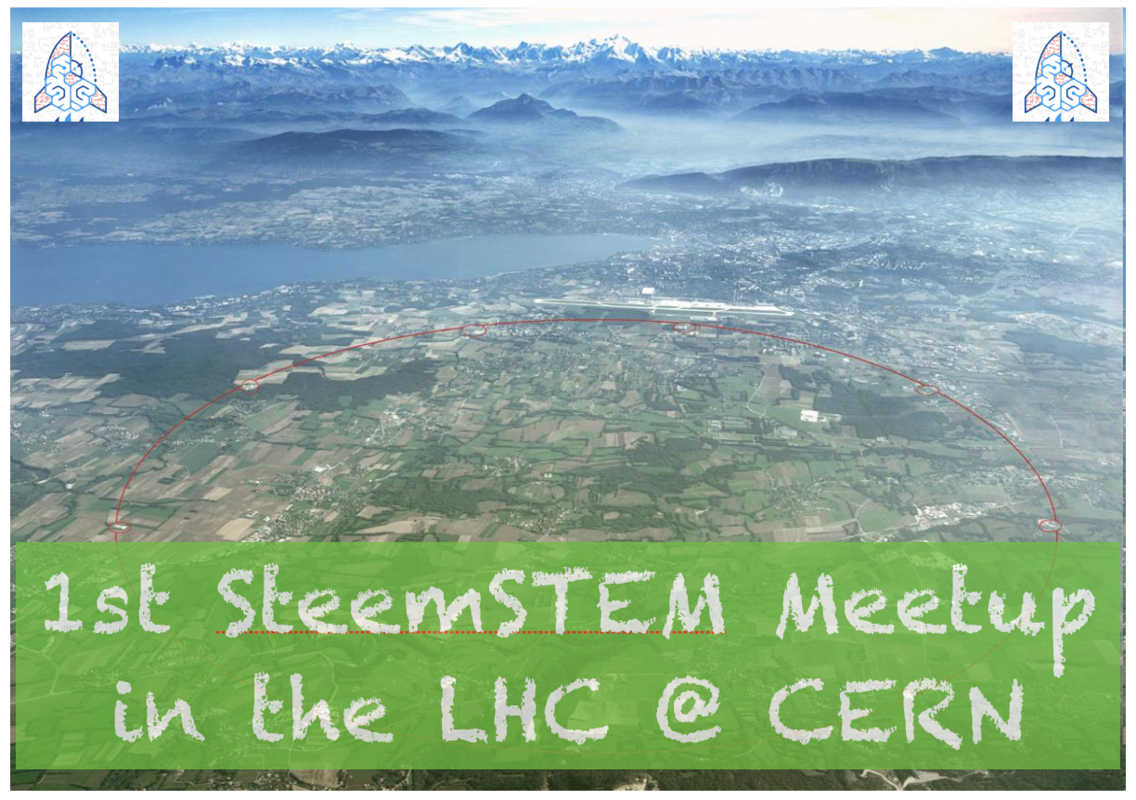
But before starting, I will quickly summarize the previous episodes of this series of posts.
- In the first episode, I discussed matter in full generality and presented how it was structured when its fundamental building blocks were scrutinized.
- In the second episode, I detailed how the fundamental forces of Nature were working, or in other words how the elementary particles were interacting with each other.
- In the episode III, I explained the needs for a Higgs boson, whose discovery has completed the list of particles expected in the Standard Model of particle physics.
- In the last episode, I finally explained how theory predictions are made in the Standard Model (and actually in any model of particle physics).
There is one fundamental question that I want to highlight today: why is the Standard Model considered as the right paradigm explaining what is going on in the microscopic world?
The answer is simple: the observations match with the theory predictions.
But then, why are researchers trying to find at all cost a more ultimate theory? This is what I want to discuss in this post.
THE STRENGTH OF THE STANDARD MODEL
As I have said just above, the Standard Model works damned well. We have an almost perfect match between theory and data.
Even after more than half a century, every single prediction turns indeed to be in full agreement with data. In addition, those predictions span a huge range in terms of energy scale. The limit on the mass of the photon is of 10-18 eV, whereas the LHC collides particles at 1013 eV.
We have 30 orders of magnitude there. I do not know any theory that does as good (if you do, please let me know).
I recall that the eV, or electronvolt, is an energy unit appropriate for the microscopic world. It is equal to 1.6 x 10-19 Joules.
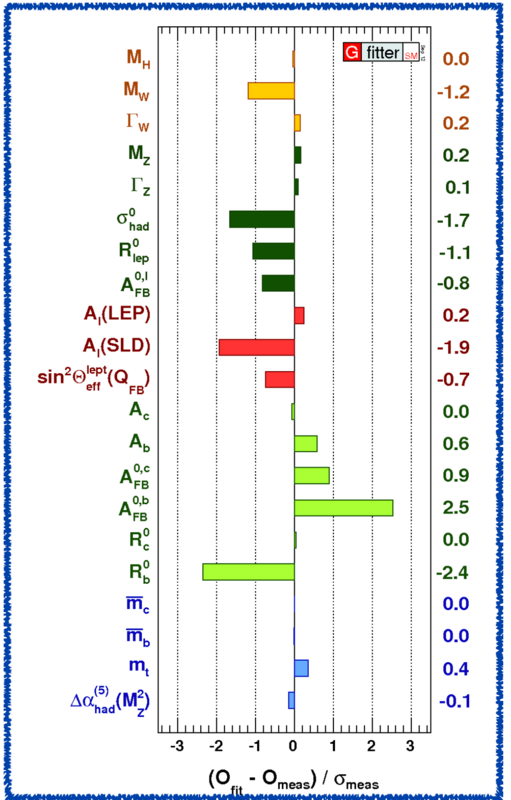
[image credits: GFitter]
Let us dig more into details the above statements. By fixing a handful of 26 parameters, thousands of predictions are found to agree with data.
A summary of some important comparisons is shown on the figure here on the right.
I know that I have already shown this figure, but it is an important one. It demonstrates this incredible level of agreement that I am not stopping mentioning since the beginning of this post.
The color rectangles show that the level of disagreement is below 3 sigmas everywhere. The x-axis indeed shows the theory-data mismatch, in terms of standard deviations (or sigmas).
In other words, this figure shows that the deviations are too small to be able claim for a hint of something new.
Another reminder: we need 3 sigmas for being allowed to mention a hint for a new phenomenon, and 5 sigmas to claim a discovery and potentially aim for a Nobel prize.
THE LIMITATIONS OF THE STANDARD MODEL AND THE LHC
The Standard Model is thus an extremely successful theory (let us use superlatives once again). Despite this success, it however features several practical limitations and conceptual issues.
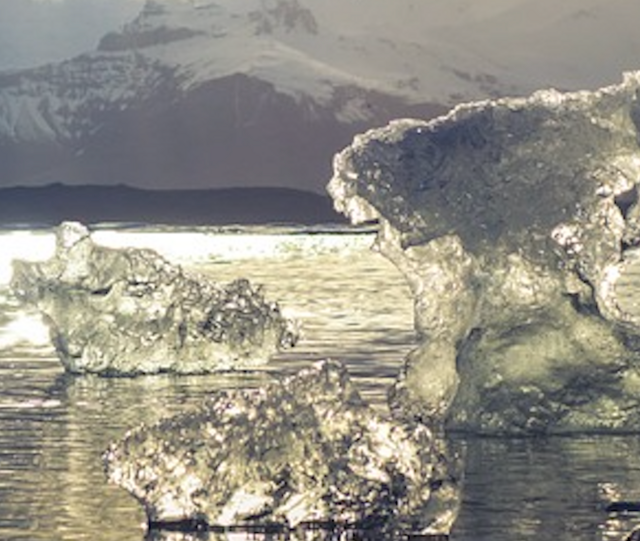
[image credits: Pixabay]
Just to name a few of them, the Standard Model has 26 parameters and this is quite a large number.
The Standard Model features a large hierarchy between the masses of the weak gauge bosons and the Planck scale (where gravity matters). This induces problems with respect to quantum corrections (and nature is quantum).
There is nothing about the neutrino masses.
There is nothing about dark matter.
And so on…
For these reasons, thousands of researchers like me acknowledge the Standard Model as a theory that consists only on the visible tip of an iceberg. And it is thus mandatory to find what is going on below sea-level.
There is in this way a more fundamental theory to be discovered, and we all have great hopes for related discoveries at the LHC. However, we must bare in mind that we may well find nothing at the LHC. We indeed have no clue about what new phenomena could be and if we have the appropriate machine to find them. But it is worth to try.
As a consequence, searches for new phenomena beyond the Standard Model (also known as new physics searches) play an important role in the LHC physics program. We want to know how our universe works after all.
In terms of new phyhsics searches, there is a huge number of them, in particular carried out by the ATLAS and CMS collaborations of the LHC. Just a reminder in passing: CMS is the detector that we will visit during our meetup.
Since we do not know the smell of new physics, we must be pragmatic and seek for every single possible option. And this is tough, on top of the fact that these new phenomena must be rare (otherwise, they should have been already discovered).
THE NEW PHYSICS CHALLENGE
Now, it is time to go back to my previous post. From a Lagrangian, we (yes you too now, with Feynman rules :p) can compute production rates for the Standard Model processes. In other words, this corresponds to the calculation of the number of collisions that will give rise to interesting Standard Model events to observe.
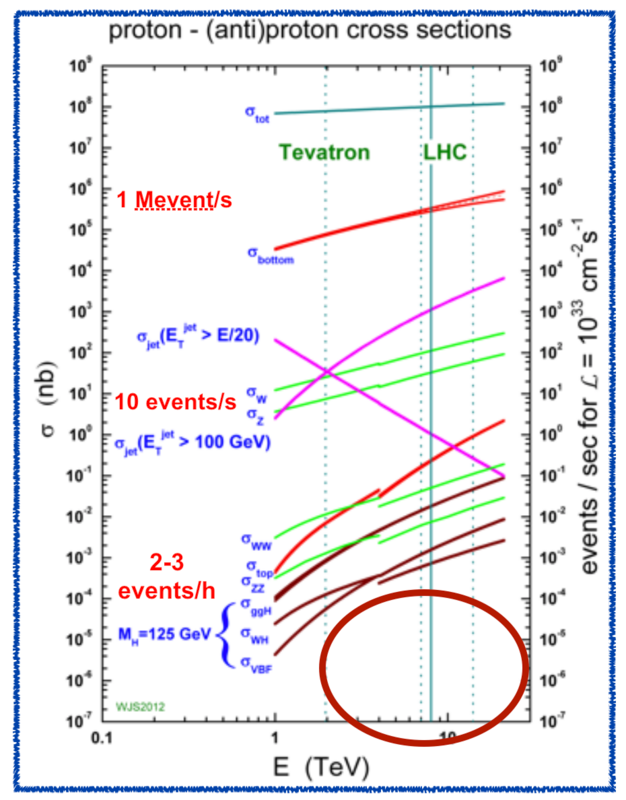
[image credits: [homemade, from now stuff available everywhere]
Those lines show the expected production rates of various Standard Model processes production rates as a function of the collision energy. The green dashed vertical line on the right corresponds to the LHC today.
The top horizontal line (with the label σtot) shows that for every second, we have about 100 millions of collisions. This is the total rate of collisions in which something happens.
However, most of these collisions are boring. One percent of them are in contrast different and more interesting (σbottom or σjet). They however only concern the strong force. We want more than just studying the strong interactions!
Now, if we want to probe weak interactions, we need to produce W bosons and Z bosons (σW and σZ). This corresponds to rates of about hundreds of events per second. Physics analyses must therefore be clever for unraveling this small number of events out of the overwhelming background made of uninteresting stuff.
The situation is even worse for the Higgs boson. We are here talking of about a few events… per hour. And for new phenomena: tens to hundreds times less than that. This is like looking for a needle… in a needle stack.
SUMMARY
With this post, I have tried to discuss a clash: the Standard Model works super well, but we now there is something more. I have discussed the reasons behind this something more, and I have tried to explain why searching for new phenomena is challenging.
The associated slides are available on my google drive. More information can be found in this textbook, among others.
In my next post, I will really discuss the LHC detectors. I just changed my mind on a last minute ;)
SteemSTEM
SteemSTEM is a community-driven project that now runs on Steemit for more than a year. We seek to build a community of science lovers on Steemit and to promote well written/informative Science Technology Engineering and Mathematics (STEM) postings in order to make Steemit a place for fascinating STEM content.
More information can be found on the @steemstem blog and on the Steemit chat and in our last project report.