One of my favorite activities on Steem is to write about my own research work. This happens every once in a while, in particular when I release one fresh paper. The topic of the day is not an exception to this.
A few weeks ago, two of my collaborators and myself published the results of a long-standing research work addressing the implications of the LHC for a new type of particles called sgluons.
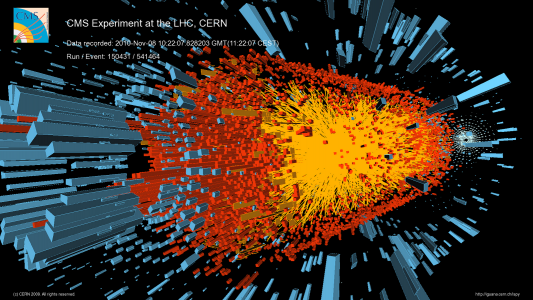
[image credits: CERN]
Those sgluon particles are not something completely crazy, popping up from nowhere. There are however several assumptions behind the prediction of their existence.
First of all, one must admit it is necessary to go beyond the Standard Model of particle physics. I will come back to this below.
Secondly, one must accept that supersymmetry is a nice idea about all the possible extensions of the Standard Model. I will also come back to this below.
Finally, as supersymmetry has not been found yet, as shown for instance here and there, supersymmetry may appear in a way that is less simple than expected. When all of this is accounted for, one ends up with non-minimal supersymmetric paradigms.
These paradigms address not only some of the problems of the Standard Model, but they also allow for evading all current constraints more easily. However, the most important point is that these non-minimal models yield new phenomena that are potentially not searched for, as not usual in more standard extensions of the Standard Model.
This was the exact point of my new research article. Some supersymmetric models are in agreement with all experimental data so far. Whilst they could be constrained or even potentially discovered with existing data, they are however not searched for in the most appropriate way.
And one must ensure that all options for new phenomena are experimentally covered to exploit the LHC as good as possible.
As usual, a stupid stuff is hidden in this post… ;)
GOING BEYOND THE STANDARD MODEL
The Standard Model is one of the most tested theory of all time. With one single theory, one covers more than 30 orders of magnitude, from the lowest experimental bound on the mass of the photon to the collision energy of the Large Hadron Collider at CERN.
After fixing a handful of parameters, one get predictions that agree with dozens of thousands of measurements undertaken during the last 60 years. Despite its success, there are however several conceptual issues with the Standard Model that make us uncomfortable.
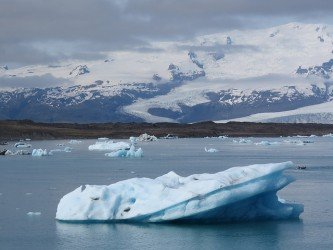
[image credits: photovicky (CC0)]
For these reasons, physicists usually consider the Standard Model as the visible part of a more fundamental theory that needs to be discovered.
In other words, the Standard Model consists in the noticeable tip of a huge and deep iceberg, and we have no clue about details of what lies below sea level.
We however have some ideas to follow. For instance, dark matter. The Standard Model does not say anything about most mass in our universe. Many extensions of the Standard Model therefore propose a candidate particle for dark matter.
Another intriguing fact comes from the Higgs boson. Whilst it allows for generating the masses of the particles of the Standard Model, the Higgs also brings a ton of others problems. The most well-known of these is the infamous hierarchy problem that many new physics theories try to solve.
And we can add many more points on this list, like neutrino masses, the unification of the fundamental interactions, the number of families in the Standard Model, etc.
As we are theoretically in the dark, the experimental program addressing the search for new phenomena is huge, and studying theoretically as many new physics theories as possible will allow us to be sure that every option will be probed.
SUPERSYMMETRY
In nature, there are two classes of particles, namely the bosons and the fermions. Bosons and fermions differ by their spin, which consequently yields these particles to have different properties. In the Standard Model, all matter particles are fermions whilst the Higgs boson and the mediators of the fundamental interactions are bosons.
Supersymmetry consists, as its name can let us guess it, in an extra symmetry of nature that one may impose when constructing a theory for the elementary particles.
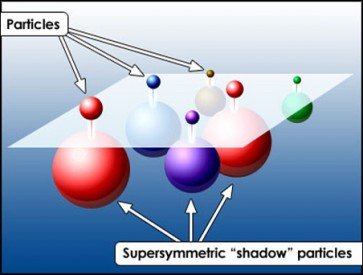
[image credits: everywhere on the web]
By definition, a supersymmetry operation changes the bosonic/fermionic nature of the particles. In other words, supersymmetry transforms a boson into a fermion, and vice versa.
In the minimal supersymmetric scenario, each Standard Model particle possesses a so-called superpartner.
We have quarks and squarks, electrons and selectrons, Higgs bosons and higgsinos, etc.
Particles connected by a supersymmetry transformation must have similar properties, like their mass, their electric charge, and so on. And here data kills us: any superpartner of the electron for instance, should have already been observed.
Supersymmetry is thus a broken symmetry, which allows for the introduction of shifts in the masses. This is not a big deal: breaking a symmetry is standard. And we get the small (the Standard Model) and big (the superpartners) spheres of the above image.
But the price to pay is that this makes a nice idea ugly… ;)
Supersymmetry has many advantages. The best ones include a solution to the hierarchy problem, an explanation for dark matter, for unification, etc.
SGLUONS, GLUONS AND GLUINOS
While supersymmetry may sound cool, there is not a single hint for supersymmetry in data. Equivalently, the preferred most minimal supersymmetric model is more and more constrained, and can only barely accommodate all data. If the simplest option fails, then one could try the next-to-simplest one.
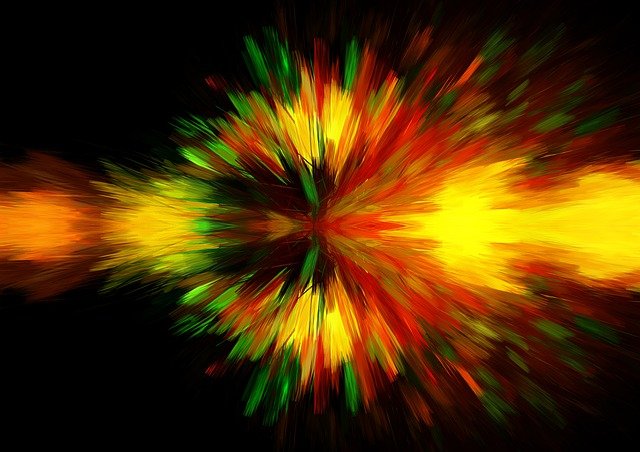
[image credits: Pixabay (CC0)]
One of the feature of the simplest superymmetric model is that the supersymmetric partner of the gluon, called the gluino, is a fermion that is its own antiparticle.
There is however no such fermions in the Standard Model, so that one may be tented to remove them.
Gluinos can have a different antiparticle, but there is a price to pay: one must add extra particles named sgluons, or scalar gluons, to the theory. Those are like gluons, but without any spin. We have two of these guys and they are technically called a scalar and a pseudoscalar sgluon (let us skip any detail here).
The scalar couples to all quarks and gluons of the Standard Model, and it is actually constrained to be very heavy. The pseudoscalar has the property to only interact with the top quark. As a result, it automatically evades all constraints stemming from current searches, as the latter assume a coupling to all quarks, to gluons, or to both.
The pseudoscalar sgluon is therefore allowed to be light, which can also be easily cooked theoretically, in contrast to the scalar guy.
CONSTRAINING PSEUDOSCALAR SGLUONS
Pseudoscalar sgluons have a specific signature made of four top quarks. The good news is that the CMS collaboration of the LHC has measured for the first time the rate associated with four-top production. Consequently, we have a handle on those pseudoscalar sgluons.
With my collaborators, we have reimplemented this CMS analysis in the MadAnalysis 5 framework, which is exactly the project I am currently running within @utopian-io (see here for the last episode and there for the main idea).
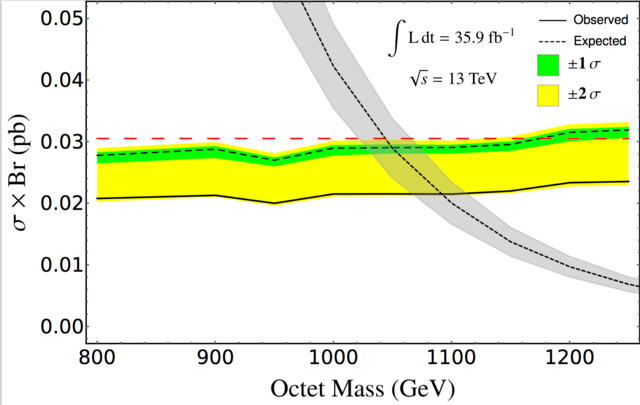
[image credits: Inspire]
Our results are shown on the so-called Brazilian plot on the right.
In grey, we have the theoretical predictions for sgluon-induced four-top production, given as a function of the mass of the sgluon
In yellow-green (the Brazilian flag), we have the rate that are maximally allowed by data for each sgluon mass.
In the region where the grey band is above the Brazilian band, we predict too many sgluons to be compatible with the non-observation of any signal. The corresponding mass hypothesis is therefore excluded.
In the region where the grey band is below the Brazilian band, sgluon-induced four-top production is small enough to be acceptable.
We have hence imposed the first constraints on pseudoscalar sgluons, that must be thus be heavier than 1 TeV (roughy 5 times the mass of the heaviest Standard Model particle).
SUMMARY
In this article, I discussed the motivation behind going beyond the Standard Model of particle physics and focused on one specific class of scenarios dubbed supersymmetry. The supersymmetric framework is extremely well motivated, but it however suffers from one important thing: no supersymmetric particle has been discovered so far.
For this reason, nature could be supersymmetric, but in fact not minimally-supersymmetric. I detailed one of my recent research work along these lines, where non-minimal supersymmetry may predict signals that are not covered by LHC searches.
One of these signals concerns pseudoscalar gluons, that were in fact only barely constrained so far. A recent CMS measurement has however changed the picture dramatically, and severe constraints now exist. In our research work,. we have quantitatively evaluated those constraints.
For the fun, we have also proposed modifications of the existing searches to make them much more efficient in probing sgluons. I hope the experimentalists will buy the idea!
STEEMSTEM
SteemSTEM is a community-driven project that now runs on Steem for more than 1.5 year. We seek to build a community of science lovers and to make the Steem blockchain a better place for Science Technology Engineering and Mathematics (STEM).
More information can be found on the @steemstem blog, on our discord server and in our last project report. Please also have a look on this post for what concerns the building of our community.