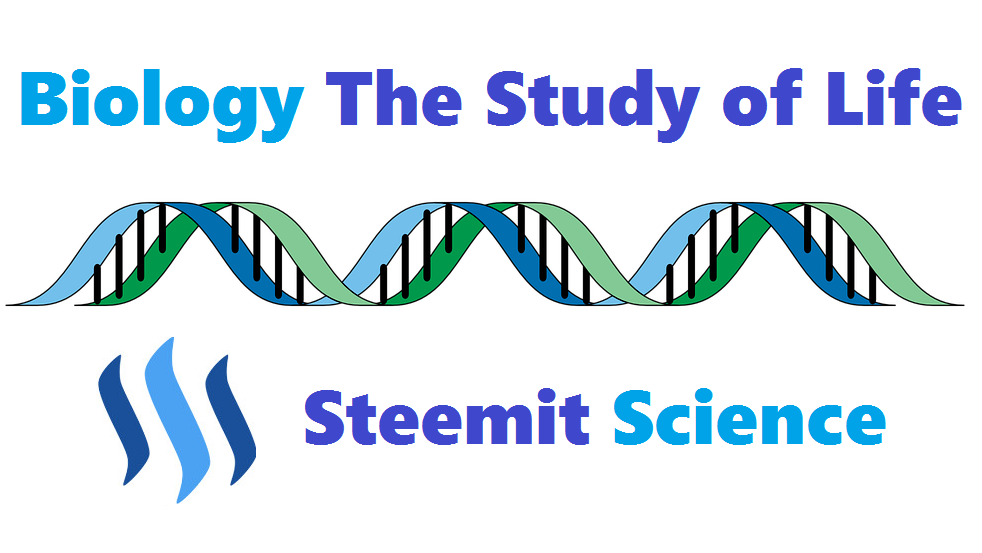
Introduction
This informative series of posts will explore modern biology; the fundamental principles of how living systems work. This material will always be presented at the level of a first-year college biology course, without assuming any prior background in biology or science. It also presents material in a conceptual format. Emphasizing the importance of broad, unifying principles, facts and details in the context of developing an overarching framework. Finally, the series takes a historical approach wherever possible. Explaining how key experiments and observations led to our current state of knowledge and introducing many of the people responsible for creating the modern science of biology.
The life of a 19th-century monk helps us to examine how genetically encoded traits are passed from parents to offspring.
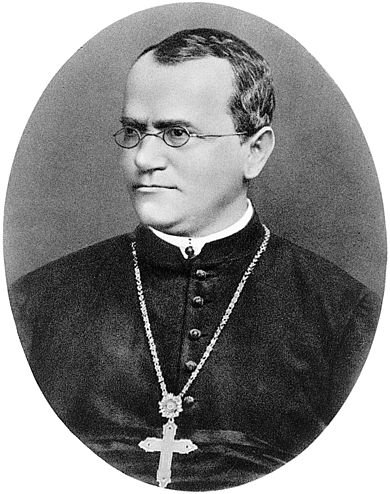
This post starts with the story of an unlikely candidate for discovering the patterns of inheritance in sexually reproducing organisms, 19th-century monk Gregor Mendel. Mendel’s experiments with pea plants in the abbey garden led to a revolution in our understanding of the role of genetics in the expression and evolution of traits in organisms. This post describes the first of Mendel’s experiments and shows how Mendel’s Law of Segregation anticipated our modern understanding of genes, chromosomes, and the formation of gametes during meiosis, even though Mendel himself had no knowledge of these phenomena.
Our modern understanding of how traits are inherited by offspring from parents began long before DNA was identified as the genetic material.
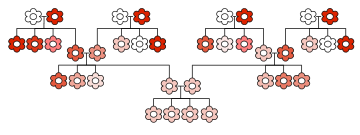
This understanding began with the work of Gregor Mendel, an Austrian monk interested in plant breeding who lived in the mid-19th century. At Mendel’s time, plant and animal breeders recognized that offspring resembled parents and used this fact to select for desirable varieties of organisms. The prevailing view, however, was that the factors responsible for inheritance simply blended together when parents mated, yielding an intermediate form. If the blending hypothesis were true, then any distinct variations among organisms should quickly vanish as the traits of sexually reproducing organisms all converged toward some mean value. Mendel realized, however, that variation is maintained in natural populations, leading him to search for an alternative way to explain patterns of inheritance.
Mendel’s experiments examined trait inheritance in garden pea plants, which proved to be advantageous experimental organisms for several reasons.
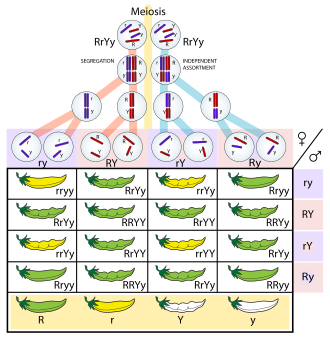
Mendel could manipulate fertilization between parental plants by cutting off their stamens (the source of pollen), then using a small brush to fertilize a plant with pollen from any other plant he chose. Pea plants also have several characteristics that occur in discrete alternative forms. For example, flower color might be purple or white or seed shape might be wrinkled or smooth, with no obvious intermediate forms. These dichotomous traits provided Mendel with a way to test the blending hypothesis explicitly.
Mendel developed “true-breeding” lines for several traits; this meant that he had breeding lines of plants that would invariably produce only one version of a character (such as all purple- or all white-flowered offspring). Mendel could assume that these true-breeding lines were uniform with respect to the factors responsible for the inheritance of the character.
Mendel’s now-classic experiment involved cross-pollinating true-breeding purple-flowered plants with true-breeding white-flowered plants.
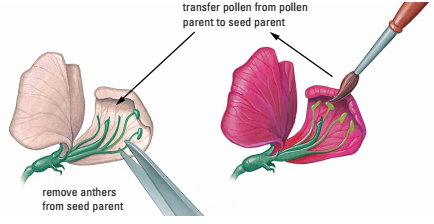
The results of this monohybrid cross were completely at odds with the prediction of the blending hypothesis. In the first (F1) generation, all offspring had purple flowers, with no evidence of inheritance of the white-flowered trait. By contrast, the blending hypothesis predicted that all offspring should have whitishpurple flowers intermediate in color from the parents.
When Mendel crossed F1 individuals to produce a second (F2) generation, he observed an even more surprising result: The offspring of this cross had either purple or white flowers, which always occurred in a 3:1 ratio. The white-flower trait that apparently had been lost in F1 individuals reemerged in some F2 individuals. Mendel found the same 3:1 ratio for all other dichotomous traits (seed color, seed shape, plant height, and so on).
To account for his observations, Mendel developed a hypothesis that described the action of genes and the division of chromosomes long before either was physically discovered.
Mendel’s hypothesis can be divided into four related ideas.
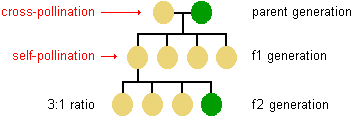
Mendel proposed a particulate “particle-based” theory of inheritance, in which different versions of “heritable factors” were responsible for inherited traits, such as flower color. We now call “heritable factors” genes and different versions of the same gene are called alleles.
Mendel also concluded that each individual must have two alleles for every gene, one from each parent.
Mendel’s third suggestion was that one allele may be dominant over other alleles. That is, if an individual possesses two alleles for the same trait, only the dominant one will have an effect. The allele that has no effect is called recessive. For example, Mendel’s F1 flowers each inherited one dominant purple-flower allele and one recessive white-flower allele; because only the dominant allele is expressed, all F1 offspring are purple.
Mendel’s fourth idea was that when a parent produces gametes, each gamete includes only one of the parent’s alleles for each gene. This has several implications. A parent with two of the same allele will necessarily produce gametes with that allele. Also, if a parent has two different alleles, 50% of their gametes will have one allele, and 50% will have the other.
Dominant and recessive alleles and the resulting patterns of offspring led Mendel to propose the Law of Segregation.
Modern terminology describes the patterns that Mendel observed.
- A homozygous individual has two of the same alleles for a given gene.
- A heterozygous individual has two different alleles for a given gene.
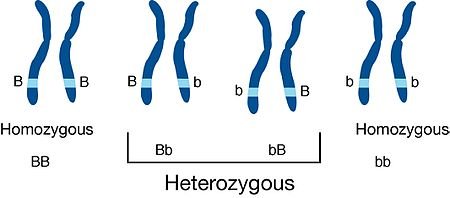
When discussing pea flowers, we will use P for the purple-color allele and p for the white-color allele.
Using upper and lowercase versions of the same letter to denote different alleles is common in genetics. Because the parent pea flowers in Mendel’s cross were homozygous and because Mendel only crossed white flowers with purple flowers, all members of the F1 generation were heterozygous. However, all F1 individuals had purple flowers. Mendel correctly argued that this was because the P allele is dominant.
Phenotypes and Genotypes
- The phenotype of an organism refers to its appearance, which results from the traits it expresses.
- The genotype of an organism refers to its genetic makeup.
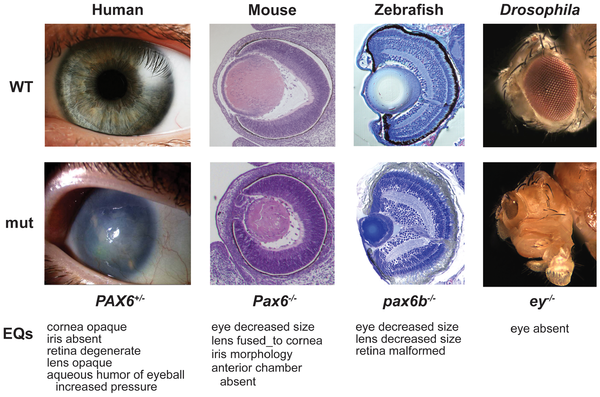
A given phenotype may be produced by more than one genotype. Because the P allele is dominant, two genotypes (PP and Pp) produce the purple-flower phenotype. However, because the p allele is recessive, only one genotype (pp) will produce the white-flower phenotype. This explains why the white-flower phenotype “disappeared” in F1; all F1 individuals had the Pp genotype. However, the allele did not vanish, and pp individuals appeared in F2.
Examining the gametes of the F1 generation explains the 3:1 ratio Mendel found.
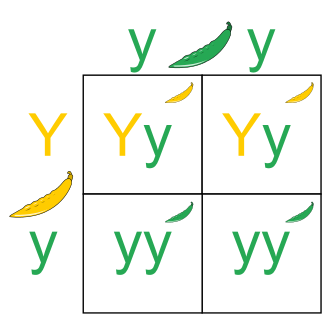
All F1 individuals were heterozygous and, thus, would produce equal numbers of P and p alleles in their gametes. The possible combinations of F1 gametes can be plotted on a Punnett square, which lists each possible allele on a row and again on a column. The boxes inside the square contain the possible genotypes. A Punnett square reveals that combining F1 gametes will produce two homozygous genotypes (PP and pp) and two copies of the heterozygous genotype (Pp). This 1:2:1 ratio (PP : Pp : pp) is expressed as a 3:1 ratio of phenotypes because the P allele is dominant and occurs in three genotypes.
Mendel’s Law of Segregation
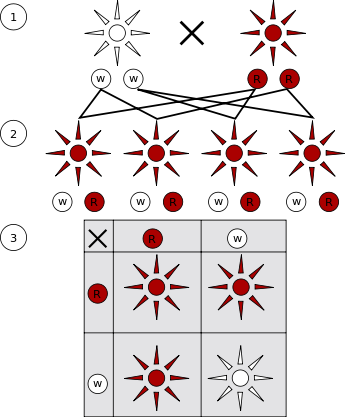
Mendel realized that the “loss” of a recessive trait and its eventual reappearance could be explained if alleles existed and if alleles separated into different gametes. The Law of Segregation not only refuted the blending hypothesis but also provided a much more comprehensive framework for examining the genetics of inheritance.
END PART 15
BIOLOGY THE STUDY OF LIFE:
PART 1 INTRODUCTION
PART 2 WHAT IS LIFE
PART 3 ORIGIN OF LIFE
PART 4 CELL TO ORGANISM
PART 5 PROTEINS
PART 6 CODE OF LIFE
PART 7 DOUBLE HELIX
PART 8 REPLICATING DNA
PART 9 CENTRAL DOGMA
PART 10 GENETIC CODE
PART 11 DNA TO RNA
PART 12 RNA TO PROTEINS
PART 13 MISTAKES HAPPEN
PART 14 WHEN CELLS DIVIDE
PART 15 MENDEL'S PEA PLANTS
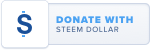



Image Credits:
ALL IMAGES UNLESS NOTED - Wikipedia