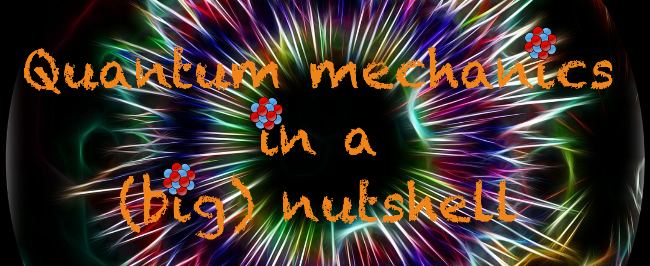
My quantum mechanics series on Steemit is probably the slowest of all my current Steemit projects. Anyways, I finally decided to go back to it and move on.
In the last two posts, I described the first models attempting to describe how atoms work. In the posts before, I introduced the fact that light is not only a wave, but can also behave like a particle (i.e. a photon).
It is now natural to dig further into quantum mechanics and write about its next very central concept: the wavy behavior of matter. Quantum mechanics does not indeed only relate waves and particles, but also relate particles and wave. Yep, it works both ways.
We are actually talking about the wave-particle duality.
THE DE BROGLIE REVOLUTION
The idea that light has both a wave nature and a particle nature is the real deal took 15 years to be fully considered by physicists. In 1923, Compton proved it experimentally once and for all: light is not only a wave but can also behave as a particle.
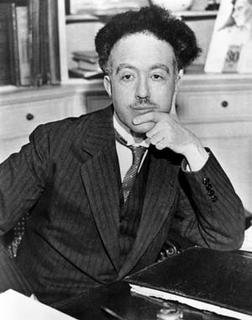
[image credits: Wikipedia]
However, at that moment, in 1923, light was the only object that was considered to have both a corpuscular and wavy side. Until a guy made a revolutionary hypothesis.
The guy is the one on the picture on the right, and his name is Louis de Broglie, to be pronounced βde Breuilβ.
The prononciation of this name is always a good way to know which students have actually attended the lectures (even for the French-speaking students)β¦
In 1923, De Broglie has proposed that a wave can be associated with the motion of any particle, and that this property does not depend on the fact that the particle is massive or massless.
Those waves are called matter waves and their existence consists of one of the cornerstones of quantum mechanics.
CHARACTERIZATION OF MATTER WAVES: WAVELENGTH
A wave is characterized by a few quantities, like its wavelength and its wavevector.
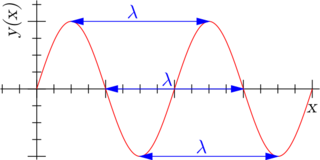
[image credits: Wikipedia]
The wavelength is what describes the spatial periodicity of the wave.
For instance, on the picture on the left (shamelessly taken from Wikipedia), the wavelength is denoted by π. This is the distance after which the wave pattern starts to reproduce itself.
One can take any point of the figure, move away from π on the x-axis, and one gets the same thing again.
In the case of the matter waves, the wavelength is called the de Broglie wavelength and is given by the ratio of the Planck constant h to the particle momentum p,
π = h / p
I recall that the momentum is a quantity mixing mass and motion, and is defined as the product of the mass of an object and its velocity.
CHARACTERIZATION OF MATTER WAVES: WAVEVECTOR
Let us now start from the above relation, after introducing the wavenumber k (that is roughly speaking the inverse of the wavelength, k = 2 Ο / π). This relation can be put into a form identical to the one derived by Einstein for light (see the next-to-last paragraph before the summary here for more information):
p = β k
where the boldface character indicates tridimensional vectors, as usual, and β is the reduced Planck constant (β= h/2Ο). One unique relation for particles and light!
This last relation tells us that the direction in which the matter wave propagates is the direction of the wavevector k, and that this direction is actually the same direction as the one of the motion of the particle (the latter is the direction of p).
Still similarly to light, one can deduce from the above relations a link between the pulsation of the wave (roughy speaking its frequency) to the energy of the particle.
In summary, de Broglie proposed to relate, to any particle in motion, a wave that propagates in the same direction as the motion of the particle.
THE EXPERIMENTAL CONFIRMATION
The assumption made by the Broglie could sound crazy. However, it was tested and confirmed. In 1927, Germer and Davisson observed interference effects typical from waves in the results of an experiment dealing with electron scattering through a crystal.
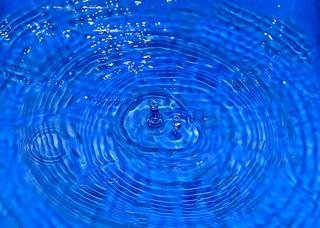
[image credits: Pixabay]
Since then, similar results have been observed for neutrons, atoms and various molecules in many many many experiments.
An interesting fact that is often unnoticed is that macroscopic objects can also be associated with a wave. The de Broglie wavelength is however so small than it is impossible to observe any visible effect that it would induce.
In order to observe matter waves, one usually builds a simple apparatus where matter waves are forced to interfere with themselves.
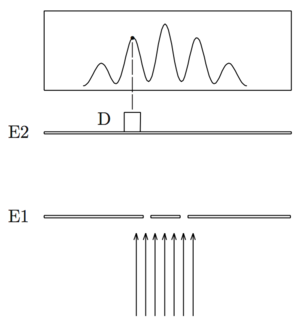
[image credits: homemade]
Such an apparatus is depicted on the picture on the left. One throws particles on a screen E1 where one has two slits. The distance between the slits must be small relatively to the de Broglie wavelength. Otherwise, we will not see anything.
The particles then pass either through the first slit, or through the second one, or they are crushed on the screen (too bad for them).
We then have a second screen E2 that is hit by the particles who passed through the slits.
A detector D can move along the E2 screen and it will count the number of particles hitting the screen at each position. We get the interference pattern shown on the above part of the picture.
DISCUSSION AND REFERENCES
Matter waves have been first theoretically proposed by de Broglie in 1923, after the corpuscular nature of light was well established. A few years alter, matter waves have been observed experimentally, and thus confirmed to be a real thing.
Interference patterns, typical from waves, have been observed many times since then, in particular when particles are sent through a double-slit system. The properties of these waves have also been found in agreement with the proposal of de Broglie.
In the next post (this one is already slightly too long I guess), I will come back to this wave-particle duality and discuss the differences between the classical physics case and the quantum mechanics case.
For more information (and references), one can check either this book, or the Wikipedia page that is not too bad.
Table of contents of this series of posts
I. Introduction
1. Concepts and fundations
2. Interactions and conservation laws
3. Systems of particles
II. The origins of quantum mechanics
1. Fundamental physics at the beginning of the 20th century
2. The mysteries of the atomic spectra
3. The mysteries of the black bodies
4. Quantization of the electromagnetic radiation and the birth of the photons
5. The Compton effect
6. The planetary model of the atom
7. The Bohr model of the atom
8. Matter waves (this post).
For more discussion on this topic (or anything related to science), please join us on steemSTEM. SteemSTEM is a community driven project which seeks to promote well written/informative Science Technology Engineering and Mathematics postings on Steemit. More information can be found on the @steemstem blog.